|
Chemical Bonds
Your feedback on these
self-help problems is appreciated. Click here
to send an e-mail.
|
Shortcut to Questions
Q: 1 2 3
4 5
6 7 8 9
10 11
|
1
|
A
chlorine atom requires one electron to attain the electronic structure
of argon (i.e. to become isoelectronic with argon)..
(1.)
Chlorine atoms can form two types of bond. What are they?
(2.)
Give examples of compounds exhibiting each of these types of bonds.
(3.)
Give an example of a polar covalent bond involving chlorine.
|
 |
2 |
Draw electron dot (Lewis) structures for the following entities.
Show all valence shell electron pairs, whether bonded or not. For
online tutorial help with this topic, see the chemcal module "Covalent
Bonding"
(1.)
HNO3 (arrangement of atoms HONO2)
(2.)
H2SO4 (arrangement of atoms (HO)2SO2)
(3.)
H3PO4 (arrangement of atoms (HO)3PO)
(4.)
SO2 (arrangement of atoms SO2)
(5.)
SO3 (arrangement of atoms SO3)
(6.)
N2O4 (arrangement of atoms O2NNO2)
(7.)
NCO- (arrangement of atoms NCO-
|
 |
3 |
A compound is formed from two elements, one of which has a high
electronegativity, and the other a low electronegativity. What properties
would the substance be expected to exhibit?
(1.)
Physical state (298 K, 101 kPa) - solid, liquid or gas?
(2.)
Melting point - high or low?
(3.)
Electrical conductivity in (a)
the solid state, (b)
the liquid state
(4.)
Solubility in water - soluble or insoluble?
(5.)
Solubility benzene - soluble or insoluble?
(6.)
Hardness - hard or soft?
|
 |
4 |
A compound is formed from two elements for which the electronegativity
difference is very small. What properties would be expected of this
substance?
(1.)
Physical state (298 K, 101 kPa) - solid, liquid or gas?
(2.)
Melting point - high or low?
(3.)
Electrical conductivity in (a)
the solid state, (b)
the liquid state
(4.)
Solubility in water - soluble or insoluble?
(5.)
Solubility in benzene - soluble or insoluble?
(6.)
Hardness - hard or soft?
|
 |
5 |
Using the information in the table classify the solids A, B, C, D as metallic, ionic, network covalent or molecular covalent:
|
A
|
B
|
C
|
D
|
Melting point (°
C) |
1700 |
800 |
80 |
1540 |
Soluble in water |
no |
yes |
no |
no |
Soluble in carbon tetrachloride |
no |
no |
yes |
no |
Solid conducts electricity |
no |
no |
no |
yes |
Fused state conducts electricity |
no |
yes |
no |
yes |
Hardness |
hard |
hard |
soft |
hard |
|
 |
6 |
(1.) The hydrogen compounds
of chlorine and sodium can be liquefied, the first by cooling, the
second by heating. Explain the reasons for the difference in melting
point of the two compounds.
(2.) Why do these liquids
differ in electrical conductivity?
|
 |
7 |
Write the appropriate word "polar", or "non-polar" for the following
molecules. For online tutorial help with this
topic, see the chemcal module "Electronegativity
and Polar Molecules"
(1.)
the carbon tetrachloride molecule (CCl4)
(2.)
a carbon-chlorine bond in the carbon tetrachloride molecule
(3.)
the 2,2-dichloropropane molecule (CH3CCl2CH3)
(4.)
boron trifluoride (BF3)
(5.)
phosphorus(V) chloride (PCl5)
(6.)
dimethyl ether (CH3OCH3)
|
 |
8 |
Indicate which of the following is a good conductor of electricity.
Write an answer for both liquid and solid sates.
(1.)
aluminium
(2.)
calcium fluoride
(3.)
carbon tetrachloride
(4.)
mercury
(5.)
phosphorus
(6.)
sulfur dioxide
|
 |
9 |
For each of the following molecules write the structural formula
and give the hybrid state of each carbon atom:
(1.) ethane (CH3CH3)
(2.) ethene (ethylene,
CH2CH2)
(3.) ethyne (acetylene,
CHCH)
|
 |
10 |
State the shape of each of the following entities (e.g. linear,
angular, trigonal planar, trigonal pyramidal, tetrahedral). For
online tutorial help with this topic, see the chemcal module "Molecular
Shape and Structure" and "Applying
VSEPR Theory"
(1.) H2O
(2.) Cl2
(3.) NH3
(4.) CH4
(5.) BF3
(6.) SO3
(7.) SO2
(8.) SO42-
(9.) H3O+
(10.) CO2
|
 |
11 |
Classify the following molecules or ions according to their shape.
(1.) PF3
(2.) SnH4
(3.) BeF42-
(4.) NO2+
(5.) GeCl2
(6.) CO32-
(7.) N2O
(8.) NO2
(9.) NO2-
(10.) OF2
(11.) SCl2
(12.) HCO2-
(13.) NCS-
|
 |
|
Answers |
|
1
|
(1.)
Chlorine forms ionic and covalent bonds.
(2.)
An example of a compound in which chlorine forms ionic bonds is
sodium chloride, NaCl(s). Sodium has a low electronegativity, whilst
chlorine has a high electronegativity. Atoms of elements which have
a large electronegativity difference tend to form predominantly
ionic bonds.
An example of covalent chlorine bonding is the bond between two
chlorine atoms in the Cl2 molecule. Here both atoms are
identical, so there is no difference in electronegativity, and the
bond is totally covalent in character.
(3.)
An example of chlorine forming polar covalent bonds is the hydrogen
chloride molecule, HCl. Here there is a small difference in electronegativity
between the Cl and H atoms. This leads to an uneven electron distribution
in the bond, and electrons will tend to spend more time near the
more electonegative element (in the case of HCl, the chlorine atom).
The polar covalent bond can be thought of as some way between a
covalent bond (where bonding electrons spend equal time around both
atoms in the bond), and an ionic bond (where bonding electrons spend
100% of the time around the more electronegative atom in the bond).
|
 |
2 |
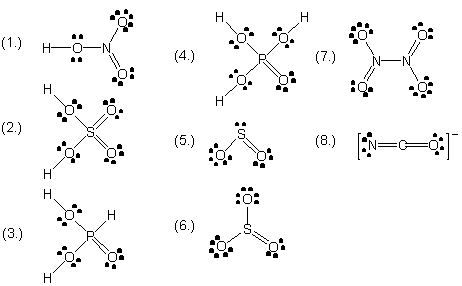 |
 |
3 |
The large difference
in electronegativity between the two elements is a strong indication
that the compound formed will exhibit predominantly ionic bonding.
This is the critical property which determines the following characteristics:
(1.)
Physical state (298 K, 101 kPa). We would expect an ionic compound
to be solid at 298K, 101 kPa.
(2.)
Melting point. The melting point of most ionic compounds is high.
The high melting point of ionic compounds (and therefore their
existence as solids at room temperature) is a direct consequence
of the very large amount of energy required to break an ion away
from the others in the crystal lattice.
(3.)(a)
Electrical conductivity in the solid state. Solid ionic compounds
are non-conductive, since the individual ions are not free to move
in the solid lattice.
(b)
Electrical conductivity in the molten (liquid) state. Molten ionic
compounds are electrical conductors. When heated to become a liquid
the solid ionic lattice breaks apart, allowing the ions to move
around in the liquid state. In this state positive ions are able
to move towards the negative electrode (and negative ions can move
towards the positive electrode) where electrolysis occurs, hence
providing a path for the conduction of an electric current.
(4.)
Solubility in water. Some ionic compounds are water soluble, whilst
others are not, so a general prediction cannot be made here.
(5.)
Solubility in benzene. Ionic compounds are generally insoluble in
non-polar solvents such as benzene.
(6.)
Hardness. Ionic compounds are hard and brittle. The strong ionic
bonds between the cations and the anions hold the ions in a very
rigid lattice. The displacement of one portion of the lattice caused
by a shock or blow results in like-charged ions being neighbours
and repelling each other, hence breaking the lattice.
|
 |
4
|
The
small difference in electronegativity between the two elements implies
that the bonding demonstrated by the compound is probably primarily
covalent in character. The following will assume that the compound
formed exhibits molecular covalent bonding (as opposed to network
covalent bonding, which is relatively uncommon and results in vastly
different properties):
(1.)
Physical state (298 K, 101 kPa). Covalent compounds can be low melting
solids, liquids, or gases at 298 K, 101 kPa.
(2.)
Melting point. Melting is the process whereby the molecules obtain
enough energy to overcome the attractive forces that hold them in
the solid state, allowing them to move about independently in the
liquid phase. Covalent compounds have low melting points (often
below room temperature). Weaker van der Waals forces operate between
component molecules instead of the strong electrostatic attractions
beteen ions that are responsible for the high melting points of
ionic compounds, so less energy is required to remove individual
molecules from a solid lattice.
(3.)(a)
Electrical conductivity in the solid state. Covalent compounds,
like ionic compounds, are electrical insulators in the solid state.
Only metals can normally behave as conductors in the solid state
due to their mobile outer electrons on each atom which readily move
from atom to atom under the influence of an electrical voltage.
(b)
Electrical conductivity in the molten (liquid) state. Covalent compounds
are electrical insulators in the liquid state. In the liquid state
a covalent compound exists as a collection of individual, neutral
molecules. Unlike the ions formed when an ionic compound melts,
covalent molecules bear no charge, and cannot act as charge carriers.
Ionic compounds when molten conduct electricity by the mechanism
of electrolysis in which cations move to the negative electrode
to receive electrons and anions move to the positive electrode to
release electrons. The flow of electrons through the external electrical
circuit constitutes an electrical current. Note that ionic compounds
undergo a chemcial reaction at each electrode in this process, so
ultimately conduction stops when all the ions have been reacted.
(4.)
Solubility in water. Covalent compounds are unlikely to be soluble,
unless they can react with the water (eg hydrogen chloride, a covalent
molecule, reacts with water to form a pair of soluble ions: HCl(g)
+ H2O(l) → H3O+
+ Cl-), or hydrogen bond with water (eg. alcohols such
as ethanol, CH3CH2OH, which are covalent molecules
containing highly polar O-H bonds capable of hydrogen bonding with
water)
(5.)
Solubility in benzene. Covalent compounds are generally soluble
in non-polar solvents such as benzene.
(6.)
Hardness. Solid covalent compounds are generally soft. The attractive
intermolecular (van der Waals) forces between the molecules in the
solid are far weaker than the electrostatic forces present between
ions in an ionic lattice, and do not require much energy to be overcome,
allowing the solid to crumble easily. In contrast, ionic compounds
are hard because of the strong attractive forces between cations
and anions. When an ionic comound does break, the planes of cations
and anions move relative to each other so that like-charged ions
become nearest neighbours, causing replusion and the crystal to
split rather than crumble - hence ionic solids are hard but brittle.
|
 |
5 |
A The
compound has a very high melting point, which indicates that it is
not a molecular covalent solid. Lack of solubility in water or carbon
tetrachloride (CCl4, an organic, non-polar solvent) also
tends to confirm that the solid is not a molecular covalent type.
The solid does not conduct electricity, which rules out metallic bonding.
The fused (molten) state is also electrically non-conductive, which
rules out ionic bonding. This leaves us with network covalent bonding,
which is also supported by the solid being described as hard and having
a very high melting point.
B
The compound has a moderately high melting point, which suggests
ionic bonding or metallic bonding. Failure to conduct electricity
in the solid state but being a conductor in the molten state indicates
the solid is ionically bonded and this is confirmed by its solubility
in water and lack of solubility in carbon tetrachloride.
C
Failure to conduct electricity in either the solid state or the
molten state rules out metallic or ionic bonding. The low melting
point strongly suggests molecular covalent bonding, and solubility
in carbon tetrachloride confirms this.
D
The ability to conduct electricity in both the solid and fused states
indicates the solid is metallic bonded.
|
 |
6 |
(1.)
The bonding in hydrogen chloride is covalent, due to the small difference
in electronegativity between hydrogen and chlorine. Therefore hydrogen
chloride has a low melting point since it is composed of discrete,
covalently bonded H-Cl molecules which are held to each other in the
solid phase by weak intermolecular forces only.
The bonding in sodium hydride is ionic due to the large difference
in electronegativity between hydrogen and sodium. Therefore sodium
hydride, NaH, has a high melting point due to the ionic bonding
throughout the lattice.
(2.)
As liquids, hydrogen chloride is a non-conductor whilst sodium hydride
conducts electricity via electrolysis due to its consisting of free,
mobile cations and anions which act as charge carriers. A voltage
applied to the molten sodium hydride results in Na+ ions
migrating towards the negative electrode, where Na(l) is then formed
according to the equation
Na+ + e- →
Na(l)
Note that at the temperature required to melt
sodium hydride, any elemental sodium formed in the electrolysis
will be in the liquid phase rather than the solid which is normal
at room temperature.] . At the positive electrode gaseous hydrogen
is formed according to
H- →
½H2(g) + e-.
From this pair of equations, the hydride and
sodium ions are seen to act as charge carriers between the two electrodes.
The result is a net movement of electrons from the positive electrode
where they are released by the hydride ions, through the external
circuit which includes the DC power source to the negative electrode,
where they are taken up by the sodium ions. Note that in the solid
state NaH(s) does not conduct current - there are ions present
in the solid, but they are fixed in the ionic lattice and
not free to travel and thereby conduct current.
The nonconductive nature of HCl(l) is due to the lack of charge
carriers. There are two ways current can be conducted through a
medium - via the movement of free electrons (as in metals) or via
movement of free ions in electrolysis (as in molten ionic compounds).
HCl(l) does not posess either of these two types of charge carriers
- all the electrons are fixed in covalent H-Cl bonds, and there
are no ions present, fixed or otherwise, so HCl(l) is a non-conductor.
|
 |
7 |
(1.)
The carbon tetrachloride molecule is non-polar. Even though the individual
C-Cl bonds are polar (chlorine is more electronegative than carbon),
the symmetry of the tetrahedral shape of the molecule means no overall
molecular polarity exists, and the molecule is non-polar. [See
the chemcal module, "Electronegativity and Polarity in Molecules"
for some 3-dimensional illustrations of this concept.]
(2.)
The individual carbon-chlorine bonds in carbon tetrachloride are
polar since chlorine is more electronegative than carbon. This means
that the bonding electron distribution in the bond is uneven, with
bonding electrons spending more time near the chlorine atom, which
takes on a partial negative charge, whilst the carbon atom takes
on a partial positive charge.
(3.)
The 2,2-dichloropropane molecule is polar since the individual carbon-chlorine
bonds and carbon-hydrogen bonds are polar. Because the shape of
the molecule is tetrahedral, the vector sum of these polar bonds
does not cancel as in the case of carbon tetrachloride above, and
results in an overall polarity in the molecule. Note that
the 2,2-dichloropropane molecule is tetrahedral about the central
atom, not planar. If the molecule were planar and the
two polar C-Cl bonds arranged opposite to each other (and thus also
the two C-H bonds), then the bond polarities would all cancel to
provide a net molecular dipole of zero. However, since the molecule
is tetrahedral, polarity of the two C-Cl bonds and the two C-H bonds
do not cancel, and a net molecular dipole results. This contrasts
with the case of carbon tetrachloride, where the molecule's tetrahedral
shape cancelled the effect of the individual polar bonds and resulted
in no molecular polarity.
(4.)
Boron trifluoride is non-polar. Due to the triangular planar shape
of the molecule with each B-F bond at 120 degrees to each other,
the individual polar boron-fluorine bonds do not result in a net
molecular polarity.
(5.)
Phosphorus(V) chloride is non-polar. The individual phosphorus-chlorine
bonds are polar, but the trigonal bipyramidal shape of the molecule
results in no net molecular polarity.
(6.)
Dimethyl ether is polar. At first glance the molecule may appear
to be linear in shape, which would result in the effect of the two
polar carbon-oxygen bonds cancelling out and no overall molecular
polarity. However, there are two lone pairs present on the central
oxygen atom which occupy two orbitals and give rise to a bent molecular
shape. As a result the polarities of the two polar carbon-oxygen
bonds partially reinforce to give a component in the same direction,
leading to an overall molecular polarity.
|
 |
8 |
(1.)
Aluminium exhibits metallic bonding, and is therefore a good electrical
conductor in both the solid and liquid state. Solid metals consist
of a lattice of atoms surrounded by a "sea" of bonding electrons
which are free to move under the influence of an applied electric
voltage, hence allowing electrons to flow through the metal. Even
in the molten state, the mobile outer electrons can move from atom
to atom when a voltage is applied to the metal and thus metals conduct
in both the molten and solid states.
(2.)
Calcium fluoride exhibits primarily ionic bonding. Individual ions
cannot act as charge carriers whilst trapped in the solid lattice
(∴ CaF2(s) is a non-conductor
of electricity). Heating the solid lattice to it's melting point
frees the Ca2+ and F- ions, allowing them
to carry charge through the liquid by electrolysis (∴
CaF2(l) is a conductor of electricity). Note the difference
between the mode of conduction by ionic compounds as compared with
metals.
(3.)
Carbon tetrachloride exists as covalent molecules. In neither the
solid nor liquid state are any free ions or electrons available
to act as charge carriers, so it is a non-conductor of electricity
in both states.
(4.)
Mercury is metallic, and is therefore a good electrical conductor
in both the liquid and solid states.
(5.)
Phosphorus exists as either single P4 molecules (white
phosphorus), or as long chains of P4 units (red phosphorus).
In both cases the bonding is covalent, and phosphorus is an electrical
non-conductor in both solid and liquid states.
(6.)
Sulfur dioxide is a covalent molecule, and, like carbon tetrachloride,
is an electrical non-conductor.
|
 |
9 |
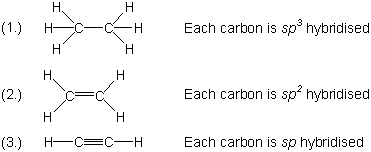 |
 |
10 |
The
shape of molecules is such that the repulsion between the atoms joined
to a central atom is minimised (ie the constituent atoms will arrange
to be as far away from one another as possible). Note that the effect
of lone pairs is critical here, as they occupy an orbital which
other atoms in the molecule will be repelled from as if they were
another bonded atom.
Since lone pairs are not bonded to a second atom, they occupy more
space than a normal bonded pair. This means that lone pairs
have a more repulsive effect on surrounding bonds than a bonded atom.
The effect is responsible for deviations from "ideal" bond angles
in molecules containing lone pairs (see below for examples of H2O
and NH3)
(1.)
The valence level of the central oxygen atom has two bonding pairs
(to H atoms) plus two lone pairs. The replusions between any four
charge clouds around a central atom is minimised if they adopt a
tetrahedral arrangement. However the shape of a molecule is defined
as the arrangement of the atoms around a central atom and the
disposition of the lone pairs is not included, even though they
have as much bearing on the molecular shape as the bonded pairs.
Thus the molecule H2O has an angular (bent) shape arising
from the two bonded pairs joining the O to the H atoms. The two
lone pairs on the O atom occupy the remaining two tetrahedrally
disposed valence level orbitals. (Without taking these lone pairs
into account it may seem that a linear shape would keep all atoms
furthest away from one another). The higher repulsive force exerted
by the O lone pairs is observed in the H-O-H bond angle. The "ideal"
bond angle in H2O is 109.5°
, which is the angle between all four orbitals in a perfect tetrahedron.
However, the measured H-O-H bond angle is reduced to 104.5°
due to the higher repulsion exerted by the two O lone pairs both
between themselves and also on the bonded pairs.
(2.)
Cl2 is linear.
(3.)
The NH3 molecule has a trigonal pyramidal shape. The
valence level of the central N atom accomodates three N-H bonds
as well as a lone pair. Four charge clouds repel each other to a
tetrahedral disposition and one of those clouds is a lone pair.
The three remaining bonded pairs would therefore have a trigonal
pyramidal shape with the N atom at the apex of the pyramid. Again,
the effect of the N lone pair is to reduce the ideal bond angle.
The repulsive force exerted by the single N lone pair is less than
that of the two O lone pairs in the water molecule example above,
and the ideal tetrahedral H-N-H bond angle of 109.5°
is only reduced to 107.3° .
(4.)
The CH4 molecule has a tetrahedral shape. The central
C atom has four electron pairs in its valence level and all are
bonded pairs, so the H-C-H bond angles are all 109.5°
.
(5.)
The shape of the BF3 molecule is trigonal planar. The
B atom has three electron pairs in its valence level. Three charge
clouds repell each other so as to have an angle of 120°
between them, and as there are no lone pairs in this molecule, its
shape will be trigonal planar.
(6.)
The SO3 molecule has a trigonal planar shape. The central
S atom has three charge clouds in its valence level and all are
bonded to O atoms. All OSO bond angles would be the ideal 120°
.
(7.)
The SO2 molecule has an angular shape. The valence level
of the central S atom has three charge clouds which are disposed
in a trigonal planar arrangement. One of these charge clouds
is a lone pair and the remaining two are used by bonding electrons
joining the S and O atoms. Thus the molecular shape is angular.
The OSO bond angle would be reduced from the ideal angle of 120°
due to the larger volume requirement of the lone pair.
(8.)
The SO42- ion has a tetrahedral shape. The
central S atom accomodates four bonds and no lone pairs.
(9.)
The H3O+ ion has a trigonal pyramidal shape.
The valence level of the central O atom has four charge clouds (therefore
arranged tetrahedrally) made up of three bonding pairs in the O-H
bonds and one lone pair. [This can be readily deduced by starting
with a water molecule and adding an H+ ion using one
of the O atom's lone pairs to form the bond]. The resultant shape
of the ion obtained by disregarding one of the corners of a tetrahedron
is a trigonal pyramid with the O atom at the apex.
(10.)
The CO2 molecule has a linear shape. The valence level
of the central carbon atom has four electrons but they are all incorporated
into the two C=O bonds and there are no remaining electrons in the
valence level as lone pairs. Consequently the molecule assumes a
linear shape (compare with SO2 and H2O where
the central atom bears one and two lone pairs, respectively).
|
 |
11 |
(1.)
The PF3 molecule has a trigonal pyramidal shape. The P
atom has five valence level electrons and each F atom provides an
additional electon to form three bonding pairs. The remaining two
electrons from the P atom make up a lone pair, providing a total of
four electron clouds around the P atom. Just like NH3 in
the previous question [N and P are in the same Periodic Table Group
and therefore have the same number of valence level electrons], the
molecular shape resulting will be trigonal pyramidal with the P atom
at the apex.
(2.)
The SnH4 molecule has a tetrahedral shape. Tin is in
the same Periodic Table Group as carbon and so has four valence
level electrons. In SnH4, each of the H atoms provides
an electron to join with one of the Sn electrons, forming a total
of four bonding pairs. There are no remaining valence electrons
on the Sn atom, so the electron arrangement and the molecular shape
of the molecule are both tetrahedral.
(3.)
The BeF42- ion has a tetrahedral shape. Beryllium
is in the second Periodic Table Group and has two valence level
electrons. Each of the F atoms provides a valence electron to form
a single bond to the Be atom and the remaining two electrons required
to make up the total of four bonding pairs come from the 2- charge
the ion carries. There are no lone pairs on the Be atom so the electron
arrangement around the Be atom and the shape of the ion are both
tetrahedral.
(4.)
The NO2+ ion has a linear shape. Nitrogen
has five valence level electrons in its atom but in this ion one
of the electrons has been lost, leaving a +1 charge on the ion.
The remaining four valence level electrons from the N atom join
with two valence level electrons from each of the O atoms to form
double bonds between the N and O atoms. As there are no lone electrons
left on the N atom, the electron arrangement around it as well as
the shape of the ion are both linear.
(5.)
The GeCl2 molecule has an angular or bent shape. Germanium
is in the same Periodic Table Group as carbon and therefore also
has four valence level electrons. In GeCl2 each Cl atom
provides one valence level electron to join with one from the Ge
atom to form a bonding pair. The remaining two valence electrons
on the Ge atom constitute a lone pair, giving a trigonal planar
arrangement of electron clouds around the Ge atom. As only the bonding
pairs are included in the shape of the molecule, GeCl2
will be angular.
(6.)
The CO32- ion has a trigonal planar shape.
There are two ways of representing this ion: either by the Lewis
diagram with with the C and O atoms joined by two single bonds plus
one double bond or (more correctly) by the molecular orbital model
where the ion is shown as containing three identical carbon/oxygen
bonds which include a delocalised pi-electron cloud encompassing
all four atoms. In either model, there are no lone electrons on
the central C atom so the distribution of charge clouds around the
C atom and the shape of the ion are both trigonal planar.
(7.)
The shape of the N2O molecule is linear. The central
N atom forms a triple bond with the other N atom and a double
bond with the O atom. This arrangement leaves no lone pairs on the
central N atom so the charge distribution and the molecular shape
are both linear.
(8.)
The NO2 molecule has an angular or bent shape. The central
N atom has five valence electrons. As for carbonate ion ((6) above)
the structure of this species can be represented by the Lewis structure
or by the molecular orbital model which incorporates a delocalised
pi-cloud of electrons encompassing all three atoms. In either version,
the N atom has three charge clouds around it - two involved in bonding
to the O atoms while a single electron resides in the third. This
unusual situation with a single lone electron localised on
the N atom still affects molecular shape in much the same way a
lone pair does. Consequently the arrangement of the charge clouds
around the N atom is trigonal planar but the shape of the molecule
is angular.
(9.)
The NO2- ion has an angular (bent) shape.
Using the model for the NO2 molecule in part (8), the
NO2- ion has just one more electron which
joins with the single lone elctron on the N atom to form a lone
pair. Consequently the shape of this ion, like the NO2
molecule, is angular.
(10.)
The OF2 molecule has an angular shape. The O atom has
six valence level electrons and each of the F atoms also provides
a single electron to form two bonding pairs. This leaves four electrons
on the O atom as two lone pairs. The four charge clouds (two bonding
pairs + two lone pairs) will adopt a tetrahedral distribution in
space but the shape of the molecule will be angular after the two
lone pairs are disregarded. Due to the larger space requirements
of lone pairs, the bonded pairs will be forced together to an angle
less than the ideal 109.5° .
(11.)
The SCl2 molecule has an angular shape. Note that as
sulfur and oxygen are in the same Periodic Table Group, the reasoning
for this part is the same as for the OF2 molecule in
part (10). Thus the S atom with six valence level electrons gains
a share of another two electrons from the two bonded Cl atoms resulting
in two bonding pairs and two lone pairs on the S atom.
(12.)
The HCO2- (formate) ion has a trigonal planar
shape. This species can also be represented by two models: (a) the
Lewis diagram showing the central C atom joined to one O atom by
a double bond and to the second O atom and the H atom by single
bonds or (b) the molecular orbital model with a delocalised pi-cloud
extending over the C and both O atoms. Either model shows three
charge clouds on the central C atom, all associated with bonding
electrons, and no lone pairs on that C atom. Thus the charge distribution
around the C atom is trigonal planar and the shape of the ion is
also trigonal planar.
(13.)
The shape of the NCS- is linear around the central C
atom. The Lewis diagram for this ion shows the central C atom joined
to the N atom by a double bond and to the S atom also by a double
bond. This accounts for all the valence level electrons on the C
atom so there are no lone pairs on it. The most stable arrangement
of two charge clouds around a central atom is linear and this is
also the shape of the ion.
|
 |
|