|
Chemical
Bonds (Advanced Questions)
Your feedback on these
self-help problems is appreciated. Click here
to send an e-mail.
|
Shortcut to Questions
Q: 1 2 3
4 5
6 7 8 9
10 11
|
1
|
Potassium and liquid bromine interact violently to produce a white
ionic solid, potassium bromide.
(1.) List the equations for the reactions
required to produce K+(g) and Br-(g) from
the solid potassium and the liquid bromine.
(2.) ΔH
for the sum of this series of reactions is +277 kJ per mole of potassium.
Does this imply that the reaction is likely or unlikely to occur?
(3.) Write the equation for the reaction
which produces potassium bromide from K+(g) and Br-(g).
(4.) Experimentally, the overall reaction
between the elements occurs exothermically. Is ΔH
for the final reaction positive or negative?
(5.) What is the name of the energy
term for the final reaction described in (3.)
Define the term named in (3.).
|
 |
2 |
What are the two extremes for the distribution of a pair of electrons
between two atoms?
|
 |
3 |
Give examples of stable molecules in which the central atom does
not have an octet of electrons (ie where the octet rule is not satisfied).
|
 |
4 |
(1.)
Diamond melts at 3773 K, while iodine melts at 387 K. Explain this
in terms of their crystal structures.
(2.) Give one further example of each
of the two kinds of crystal structure illustrated in (1.).
|
 |
5 |
Classify the dipole moments of the following molecules as either
"zero" or "nonzero". For
online tutorial help with this topic, see the chemcal module "Electronegativity
and Polar Molecules"
(1.) hydrogen iodide
(2.) carbon dioxide
(3.) ethanol
(4.) 1-chloro-2-butyne (Cl-CH2C≡CH3)
(5.) nitrogen dioxide
|
 |
6 |
Complete the following table.
Species
|
Hybridisation of central atom
|
Expected bond angle
|
Number(s) and type(s) of bonds around central atom
|
CCl4
|
sp3
|
109.5°
|
sigma bonds
|
CO2
|
sp
|
|
2 sigma and 2 pi bonds
|
HCN
|
|
180°
|
|
Cl2CO
|
|
|
approx
120°
|
NH4+
|
|
|
|
BCl3
|
|
120°
|
|
SF6
|
sp3d2
|
|
|
BeCl2
|
|
|
2 sigma bonds
|
|
 |
7 |
(1)
Complete the following table. For each of the last two columns, chose
one of the following shapes: linear, angular (bent), trigonal planar,
pyramidal, tetrahedral or none of these. For online
tutorial help with this topic, see the chemcal modules "Molecular
Shape and Structure" and "Applying
VSEPR Theory"
Molecule
|
Hybridisation of the central atom
|
Arrangement of valence shell electron
pairs
|
Shape of molecule
|
NH3
|
sp3
|
tetrahedral
|
trigonal pyramidal
|
BF3
|
|
|
|
H2O
|
|
|
|
CO2
|
|
|
|
GeCl2
|
|
|
|
(2) Which
of the five molecules in the table has the smallest angle between
the bonds joining the central atom and the remaining atoms?
(3)
List all molecules in the table which are non-polar - i.e. have
a zero dipole moment.
|
 |
8 |
Arrange the following entities in order of increasing bond angle
between a non-central atom, the central atom and a non-central atom:
carbonate ion, carbon tetrachloride molecule, water molecule, hydrogen
cyanide molecule.
|
 |
9 |
Write a suitable electron dot (Lewis) formula
for each of the following molecules. Show all valence electrons
including lone pairs. For online tutorial help with this topic,
see the chemcal module "Covalent
Bonding"
(1.)
ozone (O3)
(2.)
nitrous acid (HNO2)
(3.)
dialuminium hexachloride (Al2Cl6)
|
 |
10 |
For each of the entities listed write the structural formula (showing
lone pairs) and give the hybrid state of the selected atom(s) shown
in parenthesis beside it.
(1.) Phosphorus pentachloride molecule
(P)
(2.) Iodine pentafluoride molecule
(I)
(3.) Nitrous acid molecule (N)
(4.) Acetic (ethanoic) acid molecule
(C1 and C2)
(5.) Hydrogen peroxide molecule (O)
(6.) Hydrogen cyanide molecule (C)
(7.) Tetrafluoroborate ion (B)
(8.) Sulfur hexafluoride molecule (S)
(9.) Water molecule (O)
(10.) Dicyanoargentate ion (Ag)
(11.) Mercury(II) bromide molecule
(Hg)
(12.) Formic (methanoic) acid molecule
(C)
|
 |
|
Chemical
Bonds (Advanced) Answers
|
|
1
|
(1.)
Solid potassium vaporising and then ionising:
K(s) →
K(g)
K(g) →
K+(g) + e-
Liquid bromine (Br2(l))
vaporising, and then ionising:
½Br2(l)
→ ½Br2(g)
½Br2(g)
→
Br(g)
Br(g) + e-
→ Br-(g)
(2.)
The positive enthalpy sum for this set of reactions indicates they
require energy to proceed (ie. they are endothermic). The sign of
the enthalpy change for a reaction is not the predictor of spontaneity
for that reaction. Instead it is the sign of the free energy change,
ΔG
, which predicts the spontaneous direction of a reaction. However,
if as in this case ΔH
is large and positive,
it is less likely that these reactions as written will occur spontaneously.
(3.)
Reaction:
K+(g) + Br-(g)
→ KBr(s)
A solid lattice of K+
and Br- ions forms.
(4.)
An exothermic reaction
indicates a negative ΔH
value (ie heat is released)
(5.)
The energy released in this step is the lattice energy which
is defined as the energy required for the dissociation of one mole
of a crystal into its constituent gaseous ions. The process can
be represented by the general equation MnXy(s)
→ My+(g) + Xn-(g).
When defined in this way, energy is absorbed so the sign
of ΔH
for this process is positive. From Part (4), the energy released
when the same gas-phase ions combine to form the solid crystal is
identical in magnitude but the sign of ΔH
is negative.
|
 |
2 |
The bonding electrons
between 2 atoms can be equally shared between the two bonding atoms
on one extreme (ie a non-polar covalent bond), or completely localised
and fixed around only one atom (ie an ionic bond). However, most
bonds actually involve a degree of sharing somewhere between these
two extremes. ie all ionic bonds involve some degree of sharing,
and the only non-polar bonds in which electrons are completely evenly
shared are those between identical atoms (eg H-H).
|
 |
3 |
Some examples include:
BeCl2 (4 bonding
electrons)
BF3 (6 bonding
electrons)
PCl5 (10 bonding
electrons)
SF6 (12 bonding
electrons)
Also, many transition
metal ions do not satisfy the octet rule.
In fact, the octet rule
is only really applicable to the elements carbon →
fluorine in Period 2. In subsequent periods of the table, the availability
of d-orbitals allows atoms to hold more than 8 electrons in their
valence level. The term "expansion of the octet" is sometimes
used to describe the situation found in compounds such as PCl5
and SF6 above.
|
 |
4
|
(1.)
Diamond is an example
of a network covalent bonded solid. The strong covalent
bonding, which extends throughout the entire crystal, typically
results in solids with very high melting points.
Iodine is an example
of a molecular covalent bonded solid, in which covalent
bonds exist between the two atoms of the I2 molecule.
However, between molecules there exists only weak dispersion
forces. It is these weak dispersion forces which are overcome
when an iodine crystal is melted to release individual I2
molecules (note - not I atoms as this would require
the strong covalent I-I bonds to be broken), so the melting point
is relatively low.
(2.)
Other examples of network covalent bonding are silicon carbide (SiC),
silicon dioxide (quartz, SiO2), and the network covalent
crystalline form of boron nitride ("borazon", BN). Other examples
of molecular covalent bonding are solid CO2 and solid
O2 and most organic compounds which have low melting
points.
|
 |
5 |
The diopole moment is
a measure of the overall polarity of a molecule. A dipole moment
of zero indicates no molecular polarity, whilst increasing non-zero
values indicate increasing molecular polarity. There is a number
of factors which influence the polarity of a molecule, including
the electronegativities of atoms present and the shape of the molecule.
(Note that determining the molecular shape is very important, since
the presence of molecular symmetry can radically change the way
individual polar bonds contribute to the overall molecular polarity.
(1.)
hydrogen iodide, H-I. The electronegativity of hydrogen is 2.1 (on
the unitless Pauling scale), and that of iodine is 2.5. Therefore
iodine is more electronegative than hydrogen and we would expect
the H-I bond to be polar (ie the bonding electrons spend somewhat
more time near the more electronegative iodine atom than the less
electronegative hydrogen atom). Since the molecule is composed only
of the H-I bond we can say that there is an overall molecular polarity,
and therefore the dipole moment will be non-zero.
(2.)
carbon dioxide, CO2. The electronegativity of oxygen
is greater than that of carbon, so the C=O bond is polar. Now the
shape of the molecule must be determined. Carbon has four outer
electrons, and is involved in two C=O double bonds. Therefore the
molecular shape is linear (ie 180°
O=C=O bond angle). It
is now evident that there is no net molecular polarity since the
two polar C=O bonds points in opposite directions to each other,
and hence their contributions cancel out. The dipole moment of CO2
is therefore zero.
In contrast, SO2
(a bent, rather than linear molecule) exhibits a net
molecular dipole since the polar S=O bonds are not oriented directly
opposite each other and their bond dipoles do not completely cancel.
(3.)
ethanol, CH3CH2OH. Note the presence of an
oxygen atom which is considerably more electronegative than either
carbon or hydrogen. The oxygen atom is located at one end of the
molecule, so electron density will be higher at this end. The shape
of the molecule is angular at the C-O-H bond. Therefore the molecule
posesses a dipole moment.
(4.)
1-chloro-2-butyne, CH3C≡C-CH2Cl.
This molecule contains a polar C-Cl bond located at one end of the
molecule. There is no symmetry which cancels the polar effect out,
so there is an overall molecular symmetry, and the overall molecular
dipole moment is non-zero.
(5.)
nitrogen doxide, NO2. Nitrogen dioxide contains a polar
N-O bond and a polar N=O bond. Nitrogen posesses 5 bonding elecrons,
one of which remains unbonded in NO2 (ie a lone
electron). Lone electrons exert a repulsive effect on surrounding
bonds in much the same way as lone pairs. As a result, the O-N=O
bond angle is less than 180°,
and the polar N-O and N=O bonds create a net non-zero molecular
dipole moment.
|
 |
6 |
CO2. The central
C atom exhibits sp hybridisation, so the expected bond angle
is 180° (ie
linear).
HCN. The bond angle is
180°,
so the hybridisation of the central atom is sp. The C-H bond
is a single sigma bond, whilst the triple C≡N
bond is composed of one sigma and 2 pi bonds. This gives a total
of 2 sigma bonds and 2 pi bonds.
Cl2CO. The
bond angle is 120°,
with three atoms bonding to the central C, so the hybridisation
is sp2. There are two single C-Cl bonds and one
double C=O bond, giving a total of 3 sigma bonds and 1 pi bond.
NH4+.
The ammonium ion is a tetrahedral ion (same shape as CCl4
or CH4). There are four H atoms bonded to the central
N and no lone pairs, so the hybridisation of the N atom must be
sp3. The expected bond angle in a tetrahedral
shape is 109.5°.
All four bonds are single N-H bonds, so there are 4 sigma bonds
around the N atom. [Note that the ammonium ion is formed from an
ammonia molecule, NH3 , to which an H+ ion
is bonded on the central N atom using the lone pair of electrons
on N atom. However, once formed, all four N-H bonds are identical.]
BCl3. There
are three atoms bonded to the central B atom and no lone electrons
on that atom, so the hybridisation is sp2. All
three of these bonds are single B-Cl bonds, so there are 3 sigma
bonds present.
SF6. There
are six atoms bonded to the central S atom and no lone electrons
left, resulting in an octahedral molecular shape with the F-S-F
bond angles equal to 90°.
Each of these 6 bonds are single F-S bonds, so there are 6 sigma
bonds around the central S.
BeCl2. Be
has no lone pairs in BeCl2, so the shape will be linear
with a 180° Cl-Be-Cl
angle. The hybridisation of the Be atom is sp.
|
 |
7 |
(1.)
The hybridisation of the central atom is determined by considering
the arrangement of the valence shell - note that molecular
shape does not always determine the central atom's hybridisation,
since lone pairs will influence the hybridisation of the central
atom as well as bonding pairs because they also require an orbital.
In the example given, NH3, the valence shell arrangement
is tetrahedral, since there are three N-H bonds and a lone pair
while the shape is trigonal pyramidal as the lone pair is not counted
as part of the shape description. In summary:
Molecular shape
describes the arrangement of atoms around the central atom.
In the case of NH3 the arrangement of the three N-H bonds
is trigonal pyramidal (the N lone pair is non-bonding, and hence
not included.)
Valence shell arrangement
describes the arrangement of all electron pairs (bonding
pairs and non-bonding lone pairs) around the central atom. In the
case of NH3 the presence of the N lone pair is included
along with the 3 bonding pairs, so the arrangement of the four electron
pairs is tetrahedral.
The atom's hybridisation
is determined from the valence shell arrangement. This leads to
the assignment of sp3 hybridisation of N, based
on the tetrahedral valence shell arrangement.
BF3. There
are 3 B-F bonds present. Boron is a group 13 element and posesses
3 outer shell valence electrons. All of these 3 electrons are involved
in bonding, so there are no non-bonding electrons (ie no lone electrons
or lone pairs) present. Therefore the valence shell arrangement
and molecular shape are both trigonal planar. This leads to an sp2
assignment for the central boron atom.
H2O. There
are 2 O-H bonds present. Oxygen is a group 16 element, so it posesses
6 outer shell valence electrons. Two of these electrons are bonding
with hydrogen (1 in each of the O-H bonds), which leaves 4 electrons
not involved in any bonds. These non-bonding electrons are therefore
present as 2 lone pairs on the central oxygen atom. This leads to
a total of 4 electron pairs present on the central oxygen - 2 bonding
pairs (one pair in each O-H bond), and 2 non-bonding pairs (two
lone pairs on the O atom). Four electron pairs will arange themselves
in a tetrahedral configuration, so the valence shell arrangement
is tetrahedral. We can now assign the hybridisation of the central
O as sp3, and the molecular shape as bent
or angular (the two O lone pairs are ignored, and only the
two bonding O-H bonds are considered - they form a bent molecular
shape
BeBr2. There
are 2 Be-Br bonds present. Beryllium, a group 2 element, posesses
2 outer shell valence electrons. Both of these are involved in bonding,
so there are no non-bonding outer electrons present in the central
Be atom. Therefore the valence shell arrangement and molecular shape
are both linear. This leads to an sp hybridisation of the
central Be atom.
CO2. Carbon
is a group 14 element, and posesses 4 outer shell valence electrons.
All four of these electrons are involved in bonding to the O atoms,
each of which is joined to the C atom by one sigma and one pi bond.
Thus there are no lone electrons on the C atom and the arrangement
of the two double bonds about the central C atom is therefore linear.
The C atom is sp hybridised.
(2.)
Both bonding and non-bonding electrons exert a repulsive effect
on each other. If all bond-bond repulsions within a molecule are
equal, the bond angles will be exactly the "ideal" value - for example
the bond angle in a perfect tetrahedron is 109.5°.
Slight differences in repulsive strength result in bond angles which
deviate somewhat from this value. For similar molecules of a given
valene shell arrangement the deviation from the ideal bond angle
will be:
Lone pair - lone pair
> lone pair - bonding pair > bonding pair - bonding pair.
ie. Lone pairs exhibit
a higher repulsive force than bonding pairs.
Of the molecules listed
in the table, tetrahedral valence shell arrangements (H2O,
NH3) will have smaller bond angles (ideal bond angle
109.5°)
than either the trigonal planar (BF3, ideal bond angle
120°)
or linear (BeBr2 and CO2 ideal bond angle
180°)
arrangements.
There is one N lone pair
present in the NH3 molecule, and two O lone pairs present
in H2O. We would expect the repulsive effect of two lone
pairs to be more marked than that of one, thus H2O should
exhibit the smallest bond angle. (This is confirmed experimentally
- the measured H-N-H bond angle in NH3 is 107.3°,
whilst the H-O-H bond in H2O is 104.5°.)
(3.)
All the molecules in the table contain polar bonds (ie bonds between
elements of differing electronegativities). The molecular shape
will therefore determine the overall molecular polarity, and whether
a molecule has a dipole moment.
NH3 is trigonal
pyramidal, so the molecule is polar (ie has a net dipole moment).
BF3 is trigonal
planar, so the effect of the three polar B-F bonds cancels out,
and the molecule is therefore non-polar (ie has no dipole moment)
H2O is bent,
so there is an overall molecular polarity, and the dipole moment
will be non-zero.
BeBr2 is linear,
so the effect of the two polar Be-Br bonds cancels, and the dipole
moment is zero.
CO2 is linear,
so the effect of the two polar C=O bonds cancels, and the dipole
moment is zero.
|
 |
8 |
First we assign valence
shell arrangements:
CO32-:
trigonal planar
CCl4: tetrahedral
H2O: tetrahedral
HCN: linear
CCl4 and H2O
both have tetrahedral valence shell arrangements, but the H-O-H
bond is subject to distortion by the two O lone pairs, resulting
in a smaller bond angle than that of CCl4.
Therefore the molecules
can be arranged in order of increasing bond angle:
H2O < CCl4
< CO32- < HCN
|
 |
9 |
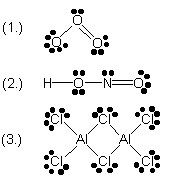 |
 |
10 |
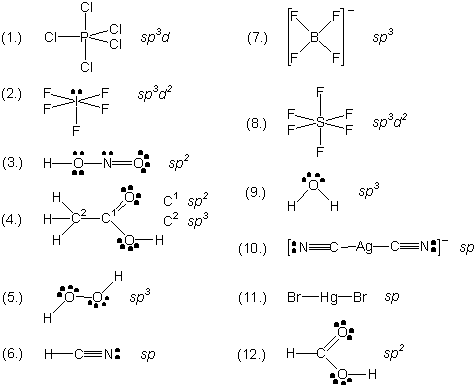 |
 |
|